The anaerobic respiration of yeast can be represented as follows:
C6H12O6 → 2C2H5OH + 2CO2 + 2ATP
In this process, glucose (C6H12O6) is converted into ethanol (C2H5OH) and carbon dioxide (CO2) while producing 2 molecules of ATP (Adenosine Triphosphate), which is the energy molecule of all organisms.
Let’s explore more knowledge, Keep Reading!
Anaerobic Respiration: Generating Energy Without Oxygen
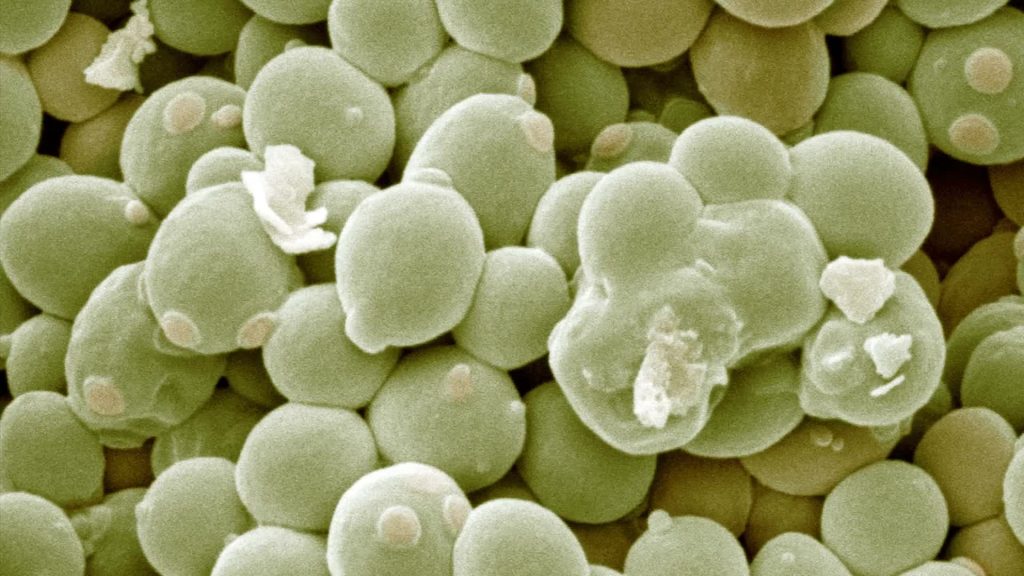
Anaerobic respiration, a biological process that unfolds in the absence of oxygen. This metabolic pathway is characterized by the breakdown of glucose into ethanol, and carbon dioxide, and the liberation of energy. It operates as a cellular respiration mechanism devoid of oxygen, serving as a vital energy source.
Moreover, this form of respiration finds application in lower organisms, such as bacteria and yeast, thriving in oxygen-deprived environments. Beyond the microbial world, anaerobic respiration comes into play during strenuous activities, like rigorous physical exercise, when the demand for energy surpasses the immediate supply of oxygen.”
Types of Anaerobic Respiration
Anaerobic respiration encompasses two primary types: lactic acid fermentation and alcoholic fermentation. These two processes occur in the absence of oxygen and serve as alternative metabolic pathways for generating energy in various organisms. Here’s a closer look at each type:
Lactic Acid Fermentation:
- Organisms: Lactic acid fermentation is commonly observed in certain microorganisms and muscle cells in animals, including humans.
- Process: During lactic acid fermentation, pyruvate, which is the end product of glycolysis, is converted into lactic acid. This conversion is carried out by enzymes, and it helps regenerate NAD+ (nicotinamide adenine dinucleotide) from NADH, allowing glycolysis to continue. This process does not release carbon dioxide.
- Applications: Also, it is responsible for the production of lactic acid in muscle cells during intense physical activity, leading to muscle fatigue and soreness. It is also used in the production of dairy products like yogurt and cheese.
Alcoholic Fermentation:
- Organisms: Alcoholic fermentation is primarily employed by certain microorganisms, such as yeast, and a few plants.
- Process: In alcoholic fermentation, pyruvate generated during glycolysis is converted into ethanol (alcohol) and carbon dioxide. This conversion involves several enzymatic reactions. The release of carbon dioxide contributes to the carbonation of alcoholic beverages.
- Applications: Further, it is a key process in the production of alcoholic beverages like beer and wine. Yeast carries out this fermentation, converting sugars into ethanol and producing the characteristic flavors and aromas associated with these beverages.
The Process of Anaerobic Respiration
There are two primary types of anaerobic respiration: lactic acid fermentation and alcoholic fermentation. Here’s an overview of the process:
Glycolysis: Anaerobic respiration begins with glycolysis, a metabolic pathway that takes place in the cytoplasm of cells. During glycolysis, a molecule of glucose (a six-carbon sugar) is broken down into two molecules of pyruvate (a three-carbon compound). This process involves a series of enzymatic reactions and yields a small amount of ATP (adenosine triphosphate) and NADH (nicotinamide adenine dinucleotide), which is an electron carrier molecule.
Fermentation:
- Lactic Acid Fermentation: The pyruvate generated during glycolysis is converted into lactic acid. This process regenerates NAD+ (nicotinamide adenine dinucleotide), allowing glycolysis to continue. During intense exercise, lactic acid can build up in muscle cells, causing discomfort and exhaustion.
- Alcoholic Fermentation: It occurs in some microorganisms, such as yeast. In this process, pyruvate is converted into ethanol (alcohol) and carbon dioxide. This type of fermentation is used in the production of alcoholic beverages like beer and wine. The release of carbon dioxide is also responsible for the carbonation in some beverages.
Energy Production: While it is less efficient than aerobic respiration (which occurs in the presence of oxygen), it does yield a small amount of ATP. The ATP generated through glycolysis helps provide short bursts of energy when oxygen is not readily available. However, the overall energy yield is lower compared to aerobic respiration.
A Brief History Of Yeast
Yeast has a long and significant history, dating back thousands of years. Ancient civilizations, such as the Egyptians, unknowingly harnessed its fermentation capabilities for bread baking and beer brewing. It wasn’t until the 17th century that yeast was first observed under a microscope by Antonie van Leeuwenhoek, though its role in fermentation remained a mystery.
Further, the breakthrough came in the 19th century when Louis Pasteur established that yeast cells were responsible for fermenting sugars into alcohol and carbon dioxide, setting the stage for modern microbiology.
Also, Pasteur’s work enabled the controlled cultivation of yeast for brewing and baking, revolutionizing these industries. In the 20th century, yeast, particularly Saccharomyces cerevisiae, became a model organism for genetic research, aiding our understanding of eukaryotic cell genetics.
Furthermore, its significance extends to biotechnology, where it is utilized to produce pharmaceuticals, biofuels, and enzymes through genetic engineering. In 2001, the Nobel Prize was awarded for yeast-related discoveries in cell cycle regulation.
Today, It remains a cornerstone in scientific, industrial, and culinary applications, reflecting our evolving knowledge of the microbial world and its pivotal role in various fields, from fermentation to biotechnology.
The Fascinating World of Yeast: From Ancient Fermentation to Scientific Discovery
In the right conditions, a single yeast cell can give birth to many generations of yeast cells. This amazing ability is why yeast is so common – it’s actually larger than bacteria, even though bacteria outnumber yeast 30 to 1.
In addition, it can ferment sugars when there’s no oxygen around. And we didn’t discover this magic; it’s been happening in nature long before humans came along. When ripe fruit falls to the ground in the jungle, yeast gets to work, turning the sugars into alcohol and carbon dioxide.
It’s no surprise that we quickly figured out how to make wine, beer, and more using this natural process. But the real game-changer was when the French scientist Louis Pasteur put yeast under a microscope and studied fermentation. He gave us a chemical equation that explained the whole process.
Yeast’s Diverse Role in Fermentation: From Ancient Origins to Modern Applications
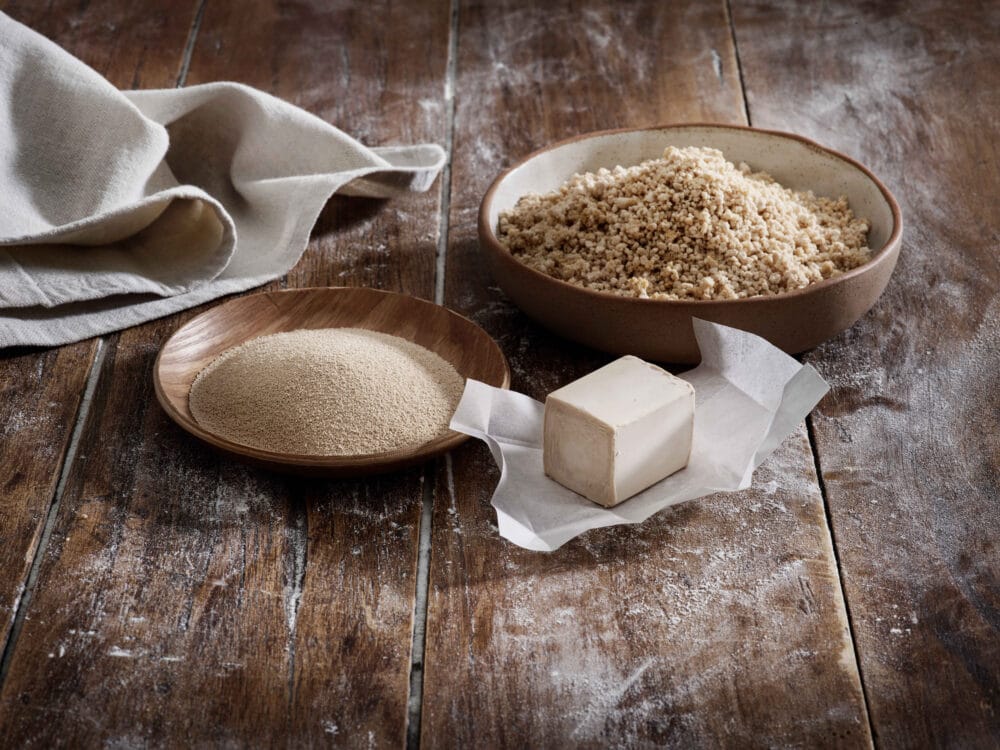
The remarkable chemistry of yeast has enabled humans to harness its transformative power for a wide array of applications, spanning the realms of food, beverages, and even renewable energy sources. Here, we delve into the fascinating applications of yeast in fermentation, including but not limited to:
1. Wine: Among the earliest products created through fermentation, wine encompasses an assortment of variations, including ciders and sake. The simplicity of fruit fermentation led to the ease of juice extraction and fermentation.
Notably, in winemaking, the carbon dioxide generated during fermentation plays a pivotal role, contributing to the effervescence of sparkling wines and aiding in the extraction of color and flavor compounds from grape skins.
2. Bread and Beer: Bread and beer, companions in human culinary history, share a common requirement for cereal grains. Once grains are roasted, the conversion of starches into sugars lures yeast for fermentation, whether it’s in bread dough or wort.
In bread, the resulting carbon dioxide is responsible for the leavening action, causing the bread to rise. In beer, carbon dioxide provides effervescence, while ethanol contributes to its alcoholic content and flavor.
3. Kombucha: Emerging around 200 BC, kombucha blended tea with fruit and sugar to entice yeast for fermentation. This complex process involves a dynamic interplay between bacteria and yeast, leading to the conversion of sugars into organic acids like acetic acid and lactic acid, as well as ethanol and carbon dioxide.
Also, the unique flavor and health benefits of kombucha are influenced by various factors, including the choice of bacterial strains, temperature, and fermentation duration. Carbon dioxide production contributes to kombucha’s natural carbonation.
4. Yogurt and Sauerkraut: Fermented foods like yogurt and sauerkraut require precision and the introduction of specific bacteria to produce lactic acid, creating their characteristic sourness and valuable probiotics. Unlike yeast-based fermentation, these foods rely on bacterial fermentation, resulting in distinct processes and outcomes.
5. Bioethanol: Bioethanol, a renewable alternative to traditional fuels, is primarily derived from corn sugar through yeast fermentation. This application emerged much later as the world sought sustainable energy sources in response to environmental concerns. Corn’s capacity to absorb carbon dioxide during growth makes it an appealing choice for addressing climate change. It’s a win-win for both energy production and environmental sustainability.
Throughout our millennia-long association with yeast, we’ve only scratched the surface of its remarkable contributions to human health, well-being, progress, and the world at large. As we continue to unlock the potential of this seemingly simple chemical equation, we eagerly anticipate the innovative ways it will continue to enhance our lives. Here’s to the exciting future that yeast holds for us all. Cheers!
Note: “In the human body, muscle tissues switch to anaerobic respiration during vigorous exercise due to the limited rate at which blood can reach these tissues. When oxygen uptake falls short of the demands for aerobic respiration, muscle cells resort to anaerobic respiration.
Besides, this metabolic shift involves the breakdown of glucose into lactic acid and the production of energy. However, the buildup of lactic acid in muscle tissues may give rise to uncomfortable muscle cramps.
FAQ’s
Which chemicals are produced in anaerobic respiration by yeast cells?
Anaerobic respiration in yeast cells produces carbon dioxide, ethanol, and energy.
What is the equation of anaerobic respiration?
The equation for anaerobic respiration is: glucose + enzymes = carbon dioxide + ethanol / lactic acid.
What is the chemical equation for yeast?
The chemical equation for yeast fermentation is: C6H12O6 (aq) → 2C2H5OH (aq) + 2CO2(g) + 2ATP.
What is anaerobic respiration in yeast called?
In micro-organisms such as yeast, the process of anaerobic respiration is called fermentation.
What is anaerobic respiration in yeast cells?
In yeast and plant cells, anaerobic respiration produces ethanol and carbon dioxide, unlike in muscle cells where lactic acid is produced.
What is the equation for anaerobic fermentation of glucose?
The equation for anaerobic fermentation of glucose is: glucose + enzymes = carbon dioxide + ethanol/lactic acid.
What is the product of anaerobic respiration?
The products of anaerobic respiration can include lactic acid in mammalian muscle cells, ethanol and carbon dioxide in yeast, and sometimes ethanol and carbon dioxide in certain plants, along with ATP.
Final Words
In summary, the chemical equation for anaerobic respiration in yeast is a remarkable illustration of how these microorganisms adapt to oxygen-deprived conditions. This process, known as alcoholic fermentation, begins with the conversion of glucose into ethanol, carbon dioxide, and a small amount of energy. Humans have harnessed yeast’s anaerobic abilities for millennia, using it to produce essentials like bread and alcoholic beverages.
Further, this equation offers a fundamental insight into the biological processes that enable yeast to produce energy without oxygen.
Understanding this mechanism has not only enhanced our culinary and industrial practices but also deepened our understanding of cellular metabolism. It underscores the adaptability of life forms and their capacity to meet essential metabolic requirements in diverse environments.